INTRODUCTION
Nonalcoholic fatty liver disease (NAFLD) is the hepatic manifestation of metabolic syndrome, and it is the most prevalent liver disease worldwide1. The prevalence of NAFLD is approximately 30% in the United States and Europe, with a similar prevalence has been documented in Asian countries2. It encompasses a spectrum ranging from simple steatosis to fatty liver with hepatocellular injury, termed nonalcoholic steatohepatitis (NASH), fibrosis and cirrhosis3. Moreover, the majority of deaths among NAFLD patients are not only associated with liver-related morbidity and mortality but also related to cardiovascular and other complications. A large number of studies have shown that high-fat diets (HFD) can cause lipid metabolism disturbances, abnormal lipid accumulation, obesity, and NAFLD 4-6. Free fatty acids (FFA) can cause oxidative stress, which is a primary cause of intravascular dysfunction. Therefore, long-term HFD can inhibit nitric oxide synthase expression in vascular endothelial cells; reduce nitric oxide (NO) production, resulting in abnormal blood vessel endothelial cell function and vascular endothelial dysfunction. NO is produced via NO synthases, which are a family of enzymes catalyzing the production of NO from L -Arginine. For this work, we will consider total nitric oxide synthase (T-NOS), endothelial nitric oxide synthase (e-NOS) and inducible nitric oxide synthase (i-NOS). T-NOS, as the name suggests, is the aggregate nitric oxide synthase (NOS) circulating at any time. At the same time, e-NOS is the endothelial NOS generated in blood vessels and is involved in the regulation of vascular function. i-NOS is inducible NOS which is usually raised in an oxidative environment. As NO expression is altered with endothelial dysfunction, which in turn is associated with NAFLD, finding an effective management solution is, therefore, a current research priority.
Previous experiments have shown aerobic exercise can improve lipid metabolism, oxidative stress 7,8 and vascular endothelial function 9. Several pharmacological and non-pharmacological strategies have been proposed to relieve NAFLD-associated deleterious alterations 10. Among non-pharmacological approaches, physical exercise-mediated multi-systemic adaptations can promote crosstalk between organs and orchestrate pro-metabolic effects known to mitigate metabolism-related disorders such as NAFLD 11. Keating et al. examined that the efficacy of commonly prescribed exercise dose and intensity for reducing liver fat and visceral adipose tissue in an animal experimental model of NAFLD, but no significant differences were found between the dose or intensity of the exercise regimen and reductions in liver fat or visceral adipose tissue 12. Paradoxically, it has been shown that vigorous and moderate exercise were equally effective in reducing intrahepatic triglyceride (TG) content, but body weight, body fat, waist circumference, and blood pressure with vigorous-moderate intensity exercise were lower than the moderate-intensity group 13,14. Similarly, Tsunoda et al. showed that vigorous intensity was more effective than moderate-low intensity exercise and moderate-high intensity protocols in preventing NAFLD from progressing to NASH 14. Two systematic reviews of published studies of NAFLD patients participating in aerobic exercise programs showed that liver fat was significantly reduced. Still, the optimal exercise intensity is undetermined 15,16, although a growing number of prospective data shows the effects ofdifferent types of exercise on NAFLD17. Collectively, these previous findings suggest that the intensity of exercise, rather than the volume or duration, may play a critical role in magnifying the protective effects against NAFLD 18.
The relationship between NAFLD and aortic endothelial function is poorly understood. It is unclear whether exercise could affect aortic endothelial function in a dietary-induced rat model of NAFLD. Moreover, different exercise intensities may produce varying effects on endothelial function in rat NAFLD models. Therefore, this study compared the impact of different exercise intensities on markers of aortic endothelial function in a HFD-induced NAFLD rat model.
MATERIALS AND METHODS
Animals
Male Sprague-Dawley (SD) rats (180-220g) were purchased from the Guangdong Medical Laboratory Animal Centre (GDM-LAC) (Guangzhou, China). According to the principles of the Helsinki declaration, this experiment was approved by the animal experiment ethics checklist of South China Normal University (iacuu-2008-0020). Rats were raised in a specific pathogen free (SPF) facilities environment (23 ± 1°C, humidity 60-70%, 12h light/dark cycle), in the Laboratory Animal Center. SD rats (n=50) were randomly divided into two experimental groups, a control group fed standard rodent chow diet (CON; n=10), and a high-fat diet group (HFD; n=40). After 16 weeks, animals that received the HFD were randomly separated into a sedentary control high fat group (HFC; n=10) or three exercise training groups: HFD and low-intensity exercise (LE; n = 10), HFD and moderate-intensity exercise (ME; n = 10), HFD and incremental intensity exercise (IE; n =10). For the next six weeks, the CON group received ad libitum feeding with standard rodent chow diet and remained sedentary, the HFD group received ad libitum feeding with a high-fat diet and remained sedentary. The LE, ME and IE groups, were fed with high a fat diet and were trained with different exercise training intensities. At the end of six weeks of treatment, the rats were sacrificed after overnight fasting. Blood samples, aorta samples, and liver samples were harvested for analysis. All procedures were performed following the “Guidelines for the Care and Use of Laboratory Animals” published by the National Institutes of Health (NIH Publication No. 85-23, revised in 1996).
Composition of the HFD
The HFD contained 5% sucrose, 18% lard, 15% egg yolk powder, 0.5% sodium cholate, and 1% cholesterol, added to the 60.5% basic standard rodent chow diet.
Exercise experimental protocol
All animals were familiarized with treadmill running (DSPT202, Qianjiang Technology Company, Hangzhou, China) at 0-15 m/min, 10-20 min per day, for six consecutive days. An electrified grid (0.6-mA intensity) was placed behind the belt of the treadmill to induce running. The rats that failed to run regularly were excluded from the training protocol. The exercise program involved 60 min/day, five days per week, for a total of six weeks. The rats performed exercises based on a protocol described previously19-21, with some modifications. The daily training intensity program was for each group respectively: LE group: 15 m/min, ME group: 20 m/min, and IE group consisted of running 10 minutes at 15 m/min, followed by a gradual increase in intensity at 20 m/min for 30 min, and increase in intensity to 27 m/min for 20 min on a motor-driven treadmill.
Outcome Measures
The primary outcome measures were the markers of oxidative stress in the aorta; T-NOS and i-NOS. superoxide dismutase (SOD), malondialdehyde (MDA), catalase (CAT), glutathione (GSH) and Total antioxidant capacity (T-AOC).
The secondary outcome measures were; the presence of aortic endothelin-1(ET-1) and e-NOS, body mass, liver mass and lipids.
We also confirmed the existence of NAFLD (liver histology) by hematoxylin and eosin (H&E) staining of embedded liver tissue samples.
Lipids
Blood samples were collected from the abdominal aorta and centrifuged at 3000 rpm for 15 min, and then serum was collected. The serum TG levels (mmol/l), total cholesterol (TC) levels (mmol/l), low-density lipoprotein cholesterol (LDL-c) levels (mmol/l), high-density lipoprotein cholesterol (HDL-c) levels (mmol/l), were detected with an automatic analyser (Toshiba AccuteTBA-40FR, Toshiba Corporation, Tokyo, Japan).
Markers of aortic oxidative stress
The aorta was removed to an ice plate, then placed in liquid nitrogen and the sample was saved for testing. Prepared fresh aorta samples were ground in saline solution to make 10% aorta homogenates, followed by centrifugation for 20 min at 4°C. The resulting supernatant was collected using specific kits according to the manufacturer’s instructions. NOS (T-NOS and i-NOS) were detected using an assay kit (Colorimetric method), (Nanjing Jiancheng Corp., Nanjing, China), NO assay kit (Nitrate reductase method).
MDA contents in the aorta were quantified using a lipid peroxidation MDA assay kit (TBA method) (Beyotime Institute of Biotechnology, Jiangsu, China) according to the manufacturer’s protocol. CAT activity assay kit (Visible light method), SOD, T-AOC activity and GSH contents were determined using a reagent kit (Colorimetric method), (Nanjing Jiancheng Corp., Nanjing, China).
Endothelin-1 and Nitric oxide synthase
ET-1 and e-NOS were measured by immune-histochemical analysis. After the abdominal cavity was opened, the aorta was wholly and quickly separated; the fixed aorta was embedded in paraffin, sliced into 5-μm- thick sections, and mounted on glass slides. The immunohistochemistry was performed with a PowerVision two-step immunohistochemistry detection kit. ET-1 and e-NOS antibodies were obtained from (Bioss Biotechnology Co., Ltd. Beijing, China). Samples were observed through JVC3-CCD camera (Nikon Corp., Tokyo, Japan), and Image-Pro Plus image (Media Cybernetics Corp., USA) processing software system was used for image acquisition and analysis. The brown granules visible in the cytoplasm or nucleus were considered the positive expression of aortic endothelial cells. The number of positive cells per section were counted in 10 random fields (400x magnification), and the percentage of positive cells (positive cells/ total cells × 100%) was calculated. Three non-consecutive sections were selected from each specimen, and those indices were averaged.
Characterization of non-alcoholic fatty liver disease
To characterize NAFLD, rat livers were fixed with 10% formalin, and the paraffin-embedded liver tissue samples were cut into 10μm sections for H&E staining. At least three randomly selected liver section images were then captured digitally (400x magnification), and each set of images was examined and photographed using nikon Eclipse Ci light microscope (Nikon Tokyo, Japan).
Statistical analysis
The statistical package for the social sciences (SPSS version 20.0, IBM Corp., USA) software was used for one-way ANOVA and Tukey’s significant difference post analysis. The Graph Pad Prism (version 5.0; Graph Pad Prism Software, La Jolla, CA, USA) software was used to draw the chart. The statistical results were expressed as means ± standard deviations (M ± SD). P value of ≤ 0.05 denoted a statistically significant difference.
RESULTS
Effect of different exercise training intensities on lipid metabolism disorders and liver histology
As shown in Fig. 1, liver histology was evaluated by H&E staining. CON group rats tissue exhibited well-arranged hepatic cords, cells with round and central nuclei, a lobular structure and an array of wheel-shaped cells along the centrilobular vein. However, in the HFC group, lipid droplets were observed in the liver sections (Fig. 1B). Lipid droplet volumes and quantities were reduced with different exercise intensities (Figs. 1C, 1D, and 1E).
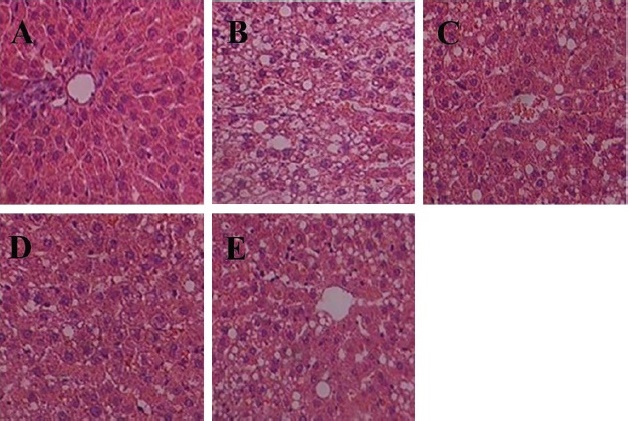
CON= control group (A); HFC= high fat control group (B); LE= HF and low intensity exercise (C); ME= HF and middle intensity exercise (D); IE= HF and incremental intensity exercise (E).
Fig. 1 The optical microscope image of H&E staining in Rat Liver Tissue (400×).
As shown in Table 1, the serum TC, TG, LDL -c and FFA were lowest in the CON group, but TC, TG and LDL -c were significantly decreased in the LE, ME and IE groups compared with the HFC group. No difference in serum HDL -c was observed between groups. Notably, TG, TC, and LDL were not significantly different between the three exercise groups.
Table 1 The change of blood lipids in each group.
Groups | TG (mmol/L) | TC (mmol/L) | LDL-c (mmol/L) | HDL-c (mmol/L) | FFA (umol/L) |
---|---|---|---|---|---|
CON | 0.45±0.13 | 1.36±0.33 | 0.26 ± 0.06 | 0.41 ± 0.01 | 313.1 ± 23.1 |
HFC | 1.55±0.21** | 5.09±0.24** | 1.88 ± 0.13** | 0.32 ± 0.07 | 627.2 ± 97.8** |
LE | 0.87±0.30##** | 4.17±0.20##** | 1.13± 0.11##** | 0.35 ± 0.11 | 569.2 ± 39.4** |
ME | 0.80±0.20##** | 4.06±0.18##** | 1.06± 0.17##** | 0.37 ± 0.08 | 558.2 ± 68.1** |
IE | 0.79±0.18##** | 4.12± 0.16##** | 1.05± 0.16##** | 0.37 ± 0.09 | 567.6 ± 49.7** |
**, P < 0.01 compared with CON group; ##, P < 0.01 compared with HFC group.
All data are expressed as mean ± SD; 8-10 animals per group were used. CON= control group; HFC= high fat control group; LE= HFD and low intensity exercise; ME=HFD and middle intensity exercise; IE= HFD and incremental intensity exercise.
Effect of different exercise training intensities on body mass and liver mass
As shown in Fig. 2, after six weeks of treatment, body mass of the rats in each group were not significantly different (Fig. 2A). Liver mass were lower in the ME group than in the HFC group (P<0.05), otherwise there was no significant difference among the exercise groups (Fig. 2B).
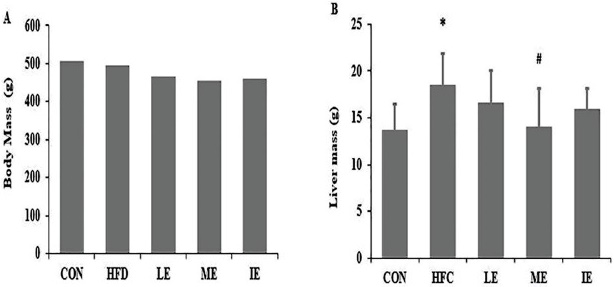
Body mass (A), liver mass (B) of each group. *, P< 0.05 compared with CON group; #, P < 0.05 compared with HFC group; CON= control group; HFC= high fat control group; LE= HF and low intensity exercise; ME= HF and middle intensity exercise; IE= HF and incremental intensity exercise.
Fig. 2 Effects of exercise training on body mass and liver mass.
Effect of different exercise training intensities on aortic endothelial cell oxidative stress
As shown in Fig. 3, compared with the CON group, CAT, GSH, and T-AOC showed a significant reduction in the HFC group (P<0.01; Fig 3C, 3D and 3E). After six weeks of exercise training, SOD, CAT, and T-AOC in LE, ME, and IE group were significantly higher compared to the HFC group (Fig. 3A, 3C and 3E), whereas the MDA levels were decreased compared to the HFC group (P<0.01; Fig. 3B). In addition, LE and ME groups exhibited significantly increased GSH compared to the HFC group (P<0.05 and P<0.01 respectively; Fig. 3D).
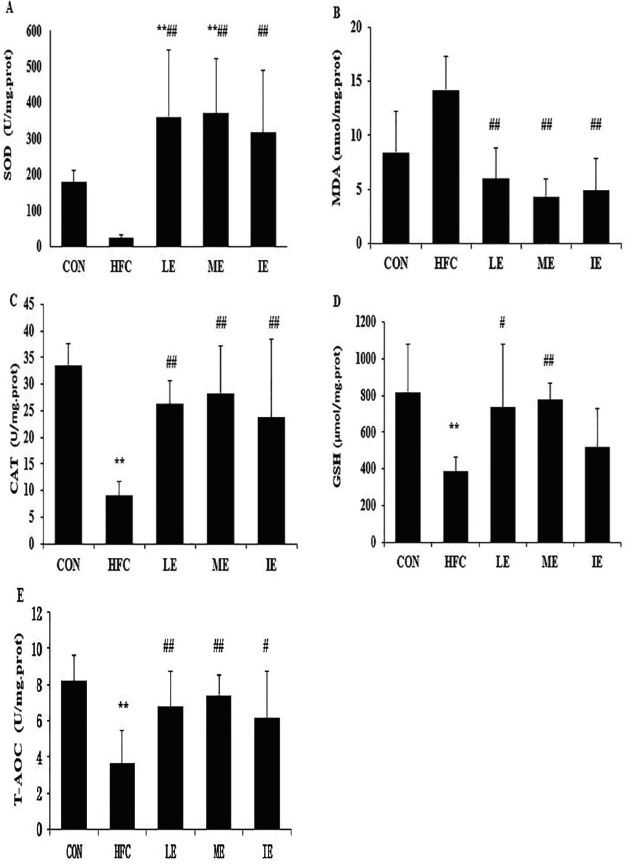
SOD (A), MDA (B), CAT (C), GST (D), and T-AOC (E). **, P < 0.01 compared with CON group; #, P < 0.05; ##, P < 0.01 compared with HFC group; CON= control group; HFC= high fat control group; LE= HF and low intensity exercise; ME= HF and middle intensity exercise; IE= HF and incremental intensity exercise.
Fig. 3 Effects of exercise training on markers of oxidative status in the aortic endothelial cell.
Effect of exercise training intensity on aortic NOS and NO activities
Table 2 shows that aortic T-NOS activity was higher in the CON versus HFC (P<0.01) and IE (P<0.05) groups; however, only the low intensity (LE) group showed a significant elevation compared to HFC (P<0.05). i-NOS activity was higher in the HFC versus CON group (P<0.01); the LE and ME groups showed a significant reduction compared to HFC (P<0.01), while the LE and ME groups showed a significant reduction compared to the IE group (P<0.01).
Table 2 The change of aortic nitric oxide synthase (NOS) activity in each group (U/mg prot).
CON | HFC | LE | ME | IE | |
---|---|---|---|---|---|
T-NOS | 6.04 ± 0.32 | 3.09 ± 0.31** | 4.79 ± 0.25# | 4.19 ± 0.31 | 4.17 ± 0.19* |
iNOS | 1.29 ± 0.14 | 2.85 ± 0.27** | 2.03 ± 0.17**##&& | 2.18 ± 0.23**##&& | 2.63 ± 0.16** |
T-NOS, Total Nitric Oxide Synthase; iNOS, inducible nitric oxide synthase; *, P<0.05; **, P<0.01 vs CON; #, P <0.05; ##, P < 0.01 vs HFC; &&, P < 0.01 vs IE.
All data are expressed as mean ± SD; 8-10 animals per group were used. CON= control group; HFC= high fat control group; LE= HFD and low intensity exercise; ME=HFD and middle intensity exercise; IE= HFD and incremental intensity exercise.
Table 3 shows that the NO content in the aorta was higher in the CON versus HFC (P<0.01) and all exercise (P<0.01) groups, all exercise groups showed a significant elevation compared to HFC (P<0.01).
Table 3 The change of aortic NO in each group (U/mg prot).
CON | HFC | LE | ME | IE | |
---|---|---|---|---|---|
NO | 202.34±11.46 | 150.21±7.72** | 176.61±9.07**## | 183.18±8.10**## | 175.43±7.02**## |
NO, Nitric Oxide ;*, P < 0.05; **, P < 0.01 vs CON; #, P < 0.05 vs HFC.
All data are expressed as mean ± SD; 8-10 animals per group were used. CON= control group; HFC= high fat control group; LE= HFD and low intensity exercise; ME=HFD and middle intensity exercise; IE= HFD and incremental intensity exercise.
Effect of different exercise training intensities on e-NOS and ET-1 expression in the aorta
The expression of e-NOS protein levels in the aorta was significantly lower in the HFC group than in CON group (P<0.05), whereas there was no difference between the three exercise groups (Table 4 and Fig. 4). The expression of ET-1 protein levels was significantly higher in the HFC, LE, and IE groups compared to the CON group. The ET-1 levels were significantly decreased by moderate-intensity exercise training (P<0.01) (Table 5 and Fig. 5).
Table 4 The change of e-NOS expression in each group.
CON | HFC | LE | ME | IE | |
---|---|---|---|---|---|
eNOS | 0.52±0.13 | 0.35±0.08* | 0.43±0.02 | 0.41±0.07 | 0.38±0.21 |
eNOS, Endothelial nitric oxide synthase; *, P < 0.05; vs CON.
All data are expressed as mean ± SD; 8-10 animals per group were used. CON= control group; HFC= high fat control group; LE= HFD and low intensity exercise; ME=HFD and middle intensity exercise; IE= HFD and incremental intensity exercise.
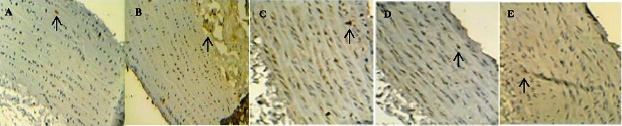
CON= control group (A); HFC= high fat control group (B); LE= HF and low intensity exercise (C); ME= HF and middle intensity exercise (D); IE= HF and incremental intensity exercise (E).
Fig. 4 Effects of exercise training on aortic e-NOS expression.
Table 5 The change of ET-1 expression in each group.
CON | HFC | LE | ME | IE | |
---|---|---|---|---|---|
ET-1 | 0.25 ± 0.03 | 0.39 ± 0.04** | 0.33 ± 0.05* | 0.29 ± 0.02## | 0.35 ± 0.09** |
ET-1, Endothelin-1; *, P < 0.05; **, P< 0.01 vs CON; ##, P < 0.01 vs HFC.
All data are expressed as mean ± SD; 8-10 animals per group were used. CON= control group; HFC= high fat control group; LE= HFD and low intensity exercise; ME=HFD and middle intensity exercise; IE= HFD and incremental intensity exercise.
DISCUSSION
The aim of our study was to investigate the effects of varying exercise intensities on aortic endothelial function in high fat diet-induced NAFLD rats. The specific process is shown in Fig 6. We can confirm that HFD induced vascular endothelial dysfunction in NAFLD rats. We found that exercise enhanced anti-oxidation function and improved some markers of aortic endothelial cell function. T-NOS activity appeared to respond best to low intensity exercise. i-NOS activity was lower only in the LE and ME groups. Moderate intensity exercise demonstrated the greatest effect on decreasing the expression of the potent vasoconstrictor ET-1 levels and the expression of NO, whereas GSH was raised in this group. Decreased blood lipids were exhibited in all exercise groups.
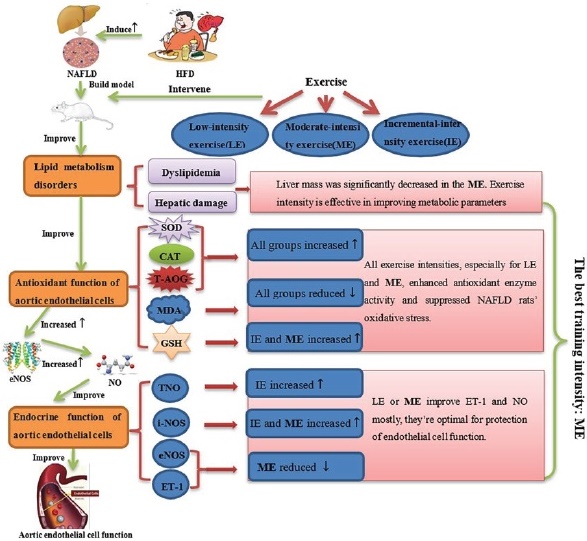
Fig. 6 Effects of different exercise intensities on antioxidant function aortic endothelial cell function and blood lipid in rats with non-alcoholic fatty liver disease.
The impact of different exercise training intensities on lipid metabolism disorders
We demonstrated that three different exercise training intensities were equally effective in alleviating dyslipidaemia as well as hepatic damage in a diet-induced rat NAFLD model. These results suggested that the therapeutic effect of exercise training in dyslipidaemia and hepatic injury is unrelated to exercise intensity. This notion is supported by meta-analytic work 22. Moreover, our study did not find any improvement in body mass or HDL -c in any group. However, it should be noted that liver mass was significantly decreased in the moderate-intensity group.
Exercise plays a vital role in improving lipid metabolism disorders and is increasingly seen as adjunctive therapy for the prevention and treatment of NAFLD16,17,23. Machado et al. showed that exercise intensity would be more effective in improving metabolic parameters than frequency or duration 24. A retrospective study indicated that moderate and vigorous-intensity physical activity yielded similar health benefits to low, in terms of the measured body adiposity and serum TG 25. Two studies showed that vigorous-intensity interval training and continuous moderate-intensity exercise have the same effect on lowering the serum FFAs, TG of NAFLD in animals 17,26. Suk et al. also indicated that high-intensity exercise improved lipid metabolism in the liver of rats27. Fisher et al. also did not find any improvements in body weights and HDL-c between groups of differing exercise intensities28. However, Khammassi et al. showed that high-intensity interval training may be particularly useful in overweight/obese youth to improve body composition and lipid profile 29.
The impact of different exercise training intensities on the antioxidant function of aortic endothelial cells
Our study showed that CAT, GSH, and T-AOC was significantly reduced in the HFC group, compared with the CON group, which confirmed the expected effect of HFD induced NAFLD rat model. After six weeks of exercise training SOD, CAT, and T-AOC were significantly increased, conversely, MDA was reduced considerably in all exercise groups. Furthermore, low intensity and moderate-intensity exercise increased GSH.
Previous work has shown that HFD increase lipid peroxidation and destroy the balance of the oxidative and antioxidative systems30. Moreover, oxidative stress and increased ROS production are the primary cause of dysfunction in aortic endothelial cells 19,20. The relationship between exercise and oxidative stress is extremely complex, depending on the mode, intensity, and duration of the exercise. Pingitore et al. noted that regular moderate training in humans appears beneficial for oxidative stress and health. Conversely, acute exercise leads to increased oxidative stress21, presumably as their period of adaptation that is missing from acute exercise training. Pereira et al. also showed high-intensity exercise might induce oxidative stress31. Li et al. reported that SOD activity and GSH were significantly raised after rats were exercised at medium intensity 32. Radak et al. also indicated that moderate exercise significantly increased the activity of antioxidant enzymes33. However, Lu et al. reported that high-intensity exercise was superior to the moderate-intensity in attenuating oxidative stress and improving glucolipid metabolism in post-MI rat myocardium34. Jamurtas et al. also found high intensity to be superior to moderate intensity for reducing oxidative stress in healthy male humans35. It, therefore, remains unclear which exercise intensity is optimal for improving antioxidant function.
In our study, we elucidated that all exercise training intensities, especially for low intensity and moderate intensity, enhanced antioxidant enzyme activity and suppressed NAFLD rats’ oxidative stress. We speculated, and our data support the notion that incremental exercise may increase reactive oxygen species (ROS) production during incremental exercise leading to the oxidation of protein, lipids or nucleic acid 36. The production of ROS during exercise is also accompanied by a reduction of antioxidant capacity 37. However, our data lack measures of ROS production and other related oxidative stress markers. So further studies of the molecular mechanisms involved in antioxidation may be indicated.
The impact of different exercise training intensities on endocrine function of aortic endothelial cells
Studies have shown that a high-fat diet can lead to lipid abnormalities, vascular endothelial damage, reduced NO content produced by endothelial cells, resulting in impaired endothelial function 38. The role of NO in the liver largely depends on the type of NOS that catalyzes its production. e-NOS plays a beneficial role in alcoholic liver disease, while i-NOS plays an important role in alcohol-induced liver damage. In the process of oxidative stress, the production of i-NOS can be induced, and a large amount of NO from i-NOS can aggravate the liver damage caused by oxidative stress, while NO produced from e-NOS can resist the effect of superoxide 39. The results of this experiment showed that compared with the CON group, the expression of ET-1 (P<0.01) in the aorta of the HFC group increased significantly, while the expression of no and e-NOS (P<0.05) decreased, and the expression of i-NOS (P<0.01) increased, suggesting that long-term high-fat diet will cause oxidative stress in rats and then lead to vascular endothelial dysfunction.
Exercise can effectively improve the function of aortic endothelial cells, but the optimal exercise intensity is still unclear. The study of Hambrecht et al. showed that exercise training, at symptom-limited intensity, improves arterial endothelial cell function in people with heart disease 40. Several previous studies have shown low and moderate-intensity exercise to have a positive effect on aortic endothelial cell function of rats 41. Shaodong et al. indicated that aerobic exercise, of unknown intensity, decreases the production of lipid oxidation products, and thus prevents damage to endothelial cells in rats with dyslipidemia42. Furthermore, a previous experiment demonstrated that moderate-intensity exercise could reduce the expression of ET-1 levels, induced by aortic injury in mice 43. Wang et al. and Archana et al. found that moderate-intensity exercise is optimal for raising serum NO 7,44. However, Morishima et al. indicated endothelial function was maintained by conducting high-intensity resistance exercise45. Meta-analysis showed that high-intensity training seems to have a superior effect on the improvement of endothelial function compared with moderate exercise in cardiac patients 46.
Our results show that although the reduction of e-NOS expression level in the ME group is not significant compared with the HFC group, it still shows a downward trend and is stronger than the IE group, with the fastest decreasing trend in the LE group. In the results of NO index, compared with the HFC group, the expression levels of NO in different exercise intensity groups were significantly increased (P<0.01), but the ME group had the greatest increase. In the experimental results of ET-1, we found that the expression levels of ET-1 in different exercise intensity groups were significantly reduced, and the ME group and IE group showed a very significant difference. At the same time, we found that compared with the IE group, the i-NOS activity of the LE group and the ME group was significantly decreased (P<0.01), which may be due to the intensity of the latter can better improve oxidative stress, leading to the reduction of i-NOS. To sum up, these results show that moderate-intensity exercise can make the most significant improvement in endothelin-1 and nitric oxide levels, which means that moderate-intensity exercise is the most ideal for protecting endothelial cell function.
Our experiments show that exercise can reduce the expression of aortic e-NOS and ET-1 protein levels, improved lipid metabolism. However, the role and underlying mechanism of exercise training in NAFLD related aortic endothelial cell function remain poorly understood. Notably, moderate intensity exercise demonstrated more effect on decreasing the expression of ET-1 protein levels, and GSH. Therefore, we believe that moderate exercise demonstrated improved aortic endothelial cell function, is underlined by the following: (i) Moderate-intensity exercise improves lipid metabolism, promoting fat mobilization and lipid energy catabolism25. (ii) Moderate-intensity exercise has a beneficial anti-oxidative effect. (iii) The reduced ET-1 and increased NO expression were significant in the ME group, ultimately improving aortic endothelial cell function47,48. We do, however, concede that results of other works are conflicting.