INTRODUCTION
According to the 2020 GLOBOCAN statistics on the incidence and mortality rates of 36 types of cancer worldwide, colorectal cancer (CRC) ranks third in terms of incidence (10.0%) but second in terms of mortality rate (9.4%) 1. Chemotherapy based on oxaliplatin (L -OHP) is a crucial component of the comprehensive treatment for CRC, which can prolong the survival of patients and improve their quality of life 2-4. However, primary and/or acquired resistance to L -OHP remains a significant factor limiting the effectiveness of CRC treatment 5,6. Therefore, it is urgent to clarify the mechanisms of L -OHP resistance in CRC and to find corresponding resistance reversal agents or chemosensitizers.
Previous studies have demonstrated that the mechanisms of resistance to platinum-based chemotherapy drugs may be related to the abnormal regulation of signalling networks within tumor cells caused by the sustained action of drugs. For example, abnormalities in signalling pathways such as PI3K/AKT and NF-κB give the cells strong anti-apoptotic abilities, leading to resistance to chemotherapy drugs 7,8. Sirtuin 3 (Sirt3) plays a crucial role in controlling physiological and pathological processes, such as regulating inflammation, oxidative stress, cell proliferation, differentiation, and apoptosis. This protein belongs to the sirtuin protein family, known for its diverse biological functions 9-11. Research has found that SIRT3 can cause mutant p53 to be deacetylated, leading to cancer cell apoptosis and increased sensitivity to chemotherapeutic drugs 12. Other evidence also suggested that SIRT3 could improve disease conditions by regulating apoptosis and utilizing the PI3K/Akt pathway in various diseases 13,14. Dihydroartemisinin (DHA) is a derivative of artemisinin, and there is evidence that DHA can act as a chemosensitizer for various cancer chemotherapeutic drugs both in vitro and in vivo15. The latest research has found that DHA can alleviate acute and chronic pain by increasing the activity of SIRT3 16. However, in CRC, no study has focused on the effect of DHA on L -OHP resistance through the SIRT3/PI3K/ AKT axis.
Based on previous studies, we hypothesize that DHA may affect the resistance of human CRC cells to L -OHP by regulating the SIRT3/PI3K/AKT axis. Our study aimed to examine the effectiveness of DHA in reversing the resistance of human colorectal cancer cells to L -OHP, as well as its underlying molecular mechanisms.
METHOD
Cell culture and induction of L-OHP resistance
Human CRC cells (LoVo, not resistant to L -OHP) were obtained from the American Type Culture Collection (CCL -229, ATCC) in Manassas, Virginia. The human L -OHP-resistant CRC cells (LoVo/L -OHP, resistant to L -OHP) were obtained by exposing LoVo cells to progressively increased concentrations of L -OHP 17. The initial concentration of L -OHP was 5 μM, and cells were exposed to L -OHP for two days in each cycle, followed by recovery in L -OHP-free culture medium. The same concentration of L -OHP was repeated for three cycles. After completing three cycles at the same concentration of L -OHP stimulation, the dose was escalated. This procedure was repeated until the final concentration of L -OHP reached 70 μM. Only cells that remained resistant to L -OHP after being cultured in an L -OHP-free medium for at least six months were used for subsequent experiments.
The LoVo/L-OHP and LoVo cells were cultured at 37°C and 5% CO2 in RPMI-1640 medium (Thermo Fisher Scientific, Inc., MA, USA) that was supplemented with 10% Gibco® fetal bovine serum (FBS) and 100 µg/mL penicillin-streptomycin. Cells were treated with PI3K inhibitor LY294002 (1μM) for 24 hours to inhibit PI3K signaling.
Preparation of Dihydroartemisinin Solution
A stock solution of dihydroartemisinin (obtained from Puyi Biological, Nanjing, China) was prepared by dissolving it in a small quantity of dimethyl sulfoxide (DMSO, procured from Sigma-Aldrich, USA). The resulting stock solution was formulated to have a concentration of either 100 or 300 nmol/L, which was sonicated and stored at 4°C. In subsequent experiments, all cell samples were treated with 30 μM L-OHP or an equivalent amount phosphate-buffered saline (PBS) for treatment.
Cell transfection
The siRNA targeting the SIRT3 gene (si-SIRT3#1, #2, #3) and the negative control (NC) were procured from GeneChem Corp. for cell transfection experiments.
According to the manufacturer’s instructions, the LoVo/L -OHP cells were transfected with 5 nM of the aforementioned si-RNA or negative controls. Transfection was carried out by using the lipofectamine 3000 (Invitrogen) reagent, following the prescribed protocol, while incubating the cells in a serum-free Opti-MEM medium. After 48 hours of transfection, the transfection efficiency was evaluated through RT-qPCR.
Cell Counting Kit-8 (CCK-8)
LoVo, LoVo/L -OHP, and LoVo/L -OHP+DHA (100nmol/L or 300nmol/L) cells were treated with different concentrations of OHP (5μM-70μM) 17,18. Cells were cultured into 96-well plates at 5×103 cells/ well density. After incubating the cells with CCK-8 reagent (10μL, Sangon) for 2 hours, the absorbance at 450 nm was determined on a microplate reader (Thermo Fisher Scientific, MA, USA). The half-maximal inhibitory concentration (IC50) of L-OHP was determined in LoVo and LoVo/L-OHP cells 19.
Reverse transcription-quantitative polymerase chain reaction (RT-qPCR)
RT-qPCR was used to determine the expression of PI3K, SIRT3 and AKT in the cells. We utilized the RNAiso plus kit (procured from Takara, Japan) to obtain RNA from cells and subsequently performed reverse transcription into cDNA using the PrimeScriptTM one-step qRT-PCR kit (obtained from Takara, Dalian, China). For RT-qPCR, we employed the SYBR Premix ExTaq (TaKaRa) and the ABI PRISM7300 Sequence Detection System (from Applied Biosystems). Primer sequences are shown in Table 1. GAPDH served as an internal control. The mRNA expressions were calculated by the 2-ΔΔCt method.
Table 1 The sequences of all constructs.
Name | Sequence |
---|---|
SIRT3 | Forward 5’-CCCCAAGCCCTTTTTCACTTT-3’ |
Reverse 5’-CGACACTCTCTCAAGCCCA-3’ | |
PI3K | Forward 5’-TCTAAACCCTGCTCATC-3’ |
Reverse 5’-CTTGCCGTAAATCATCCC-3’ | |
AKT | Forward 5’-CTACAACCAGGACCATGAGAAG-3’ |
Reverse 5’-ACACGATACCGGCAAAGAAG-3’ | |
GAPDH | Forward 5′-CACCCACTCCTCCACCTTTG-3′ |
Reverse 5′-CCACCACCCTGTTGCTGTAG-3′ |
Western blot
Total proteins were extracted from the cell lysates and quantified using the Pierce™ BCA Protein Assay Kit (Thermo Scientific). Then, 20 μg of the extracted proteins were separated by SDS-PAGE and transferred onto PVDF membranes. The PVDF membranes were then incubated with a primary antibody at 4˚C overnight. After washing, the membranes were incubated with Goat Anti-Rabbit IgG H&L secondary antibody (ab96899, 1/1000) at 37˚C for 45 minutes. The primary antibodies included the SIRT3 antibody ab217319, 1/1000, Abcam), PI3K antibody (ab140307, 1/2000, Abcam), AKT antibody (ab8805, 1/1000, Abcam), bcl-2 antibody (ab32124, 1/1000, Abcam), Bax antibody (ab32503, 1/1000, Abcam), cleaved caspase-3 antibody (ab32042, 1/5000, Abcam), P-gb (ab202976, 1/1000, Abcam), GAPDH antibody (ab8245, 1/1000, Mybiosource), and GAPDH was served as an internal control. We utilized the Pierce ECL Western Blotting Substrate to visualize the protein bands, which was procured from Pierce in Shanghai, China. The membranes were developed and imaged using a chemiluminescence imaging system.
Flow cytometry for cell apoptosis
Cells were incubated at 37°C with 5% CO2. After the incubation of 24 h, LoVo/LOHP cells were collected into 1.5 mL tubes with annexin-FITC and propidium iodide (PI) regents, followed by incubation for 10 min. To detect cell apoptosis, 200 μL PI was supplemented with 1 mL phosphate buffer solution (PBS) into the flow tube. Then, the apoptotic cells were measured using flow cytometry (Beckman Coulter).
RESULTS
DHA inhibited the proliferation of LoVo/ L-OHP cells and induced their apoptosis
We first treated LoVo/L-OHP cells with different concentrations (100 nmol/L or 300 nmol/L) of DHA then used the CCK-8 assay to detect the IC50 of all cells. As shown in Fig. 1-A, the IC50 of LoVo/L-OHP cells to L-OHP remarkably declined (p<0.05) after DHA treatment. In the next experiment, we treated LoVo/L-OHP cells with 30μM L-OHP or an equal amount of phosphate-buffered saline (PBS) and investigated the changes in cell viability of LoVo/L-OHP cells after DHA treatment. Our findings demonstrated a notable decline in cell viability of LoVo/L-OHP cells following DHA treatment (p<0.05, as depicted in Fig. 1-B). Moreover, flow cytometry analysis substantiated a marked rise in apoptotic cells in LoVo/L-OHP cells when treated with 300 nmol/L DHA (p<0.05, as illustrated in Fig. 1-C). Furthermore, compared with the LoVo/L-OHP group, the expression of cleaved caspase-3 protein and the Bax/Bcl-2 ratio in the L-OHP +300 nmol/L DHA and L-OHP +100nmol/L DHA groups were remarkably increased, while the expression of P-gb protein was markedly decreased (p<0.05, Fig. 1-D). In the subsequent experiments, cells were treated with 300 nmol/L of DHA.
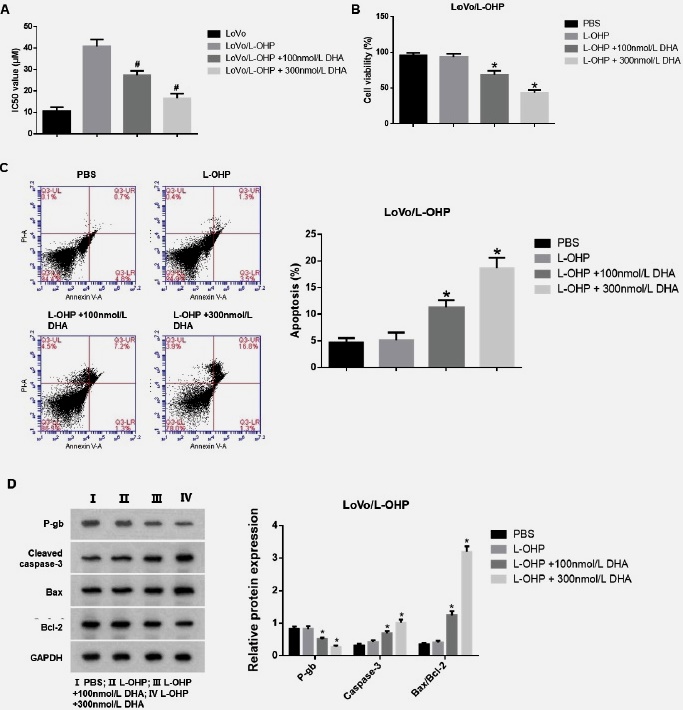
Fig. 1 DHA inhibited the proliferation of LoVo/L-OHP cells and induced their apoptosis. (A) The IC50 of all groups of cells were detected by CCK-8 assay. (B) Cell viability of all groups of cells was detected by CCK-8 assay. (C) The apoptosis rate of all groups of cells was analyzed by flow cytometry. (D) The expression of P-gp protein related to drug resistance and apoptosis-related proteins, cleaved caspase-3 protein, Bax, and Bcl-2, was detected by Western blot. Continuous data were compared using ANOVA. *p<0.05 compared with the L-OHP group. #p<0.05 compared with the LoVo/L-OHP group.
The expression of SIRT3, PI3K, and AKT in LoVo/L-OHP cells after DHA treatment
To further investigate the expression of SIRT3, PI3K, and AKT in LoVo/L-OHP cells after DHA treatment, we examined the expression of SIRT3, PI3K, and AKT in each group of cells using WB and PCR. As shown in Figs 2 A-B, our results indicated a significant decrease in SIRT3 expression level, whereas PI3K and AKT expression levels were significantly elevated in LoVo/L-OHP cells compared to the LoVo group (p<0.05). After administering L-OHP to LoVo/L-OHP cells and subsequently treating them with 300 nmol/L DHA, we observed a noteworthy upsurge in the protein and mRNA levels of SIRT3 as compared to the LoVo/L-OHP+L-OHP group (p<0.05). Furthermore, we observed a reduction in expression levels of PI3K and AKT in cells following DHA treatment (p<0.05).
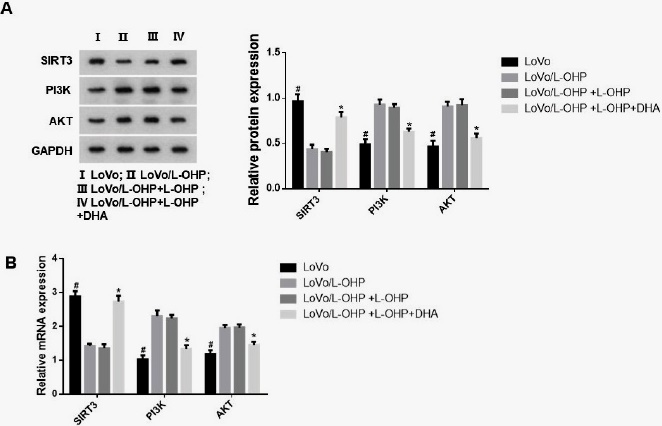
Fig. 2 The expression of SIRT3, PI3K and AKT in LoVo/L-OHP cells after DHA treatment. The SIRT3, PI3K and AKT expression in each group were measured by WB (A) and PCR (B). ANOVA was used to compare the groups. #p<0.05 compared with the LoVo/L-OHP group. *p<0.05 compared with the LoVo/L-OHP+L-OHP group.
Silencing SIRT3 promoted L-OHP resistance in LoVo/L-OHP cells after DHA treatment through the PI3K/AKT pathway
To validate the role of the SIRT3/PI3K/AKT signalling pathway in L -OHP resistance of LoVo/L -OHP cells after DHA treatment, we transfected cells with si-SIRT3#1, si- SIRT3#2, si-SIRT3#3, and si-NC and measured the knockdown efficiency of SIRT3. As shown in Figs. 3 A-B, in LoVo/L -OHP cells after DHA treatment, si-SIRT3#1, si-SIRT3#2, and si-SIRT3#3 all markably reduced the expression of SIRT3, and we selected si-SIRT3#2 with the highest knockdown efficiency for subsequent experiments. In addition, we also used the PI3K inhibitor LY294002 to inhibit the PI3K/AKT pathway and divided the cells into groups: conctrl (LoVo/L -OHP+L -OHP+DHA), si-NC (conctrol+si-NC), si-SIRT3 (conctrol+si- SIRT3), and si-SIRT3+LY294002 (conctrol+ si-SIRT3+LY294002). As shown in Fig. 3-C, compared with the si-NC group, silencing SIRT3 remarkably increased cell viability and reduced the proportion of cell apoptosis (p<0.05). However, the addition of the PI3K agonist LY294002 reversed this effect. Compared with the si-SIRT3 group, the si- SIRT3+LY294002 group had significantly reduced cell viability and increased apoptosis (Fig. 3-D).
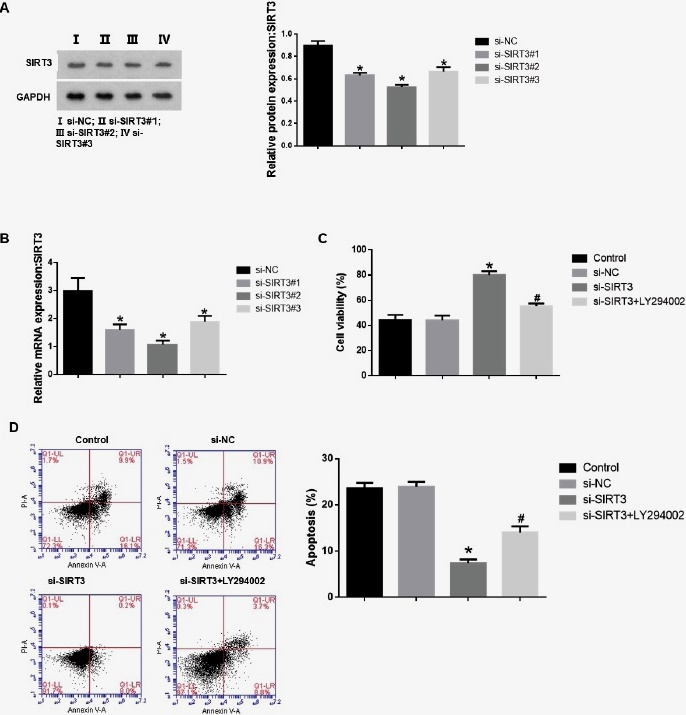
Fig. 3 Silencing SIRT3 promotes L-OHP resistance in LoVo/L-OHP cells after DHA treatment through the PI3K/AKT pathway. The expression of SIRT3 was measured by WB (A) and PCR (B). (C) Cell viability of all groups of cells was detected by CCK-8 assay. (D) The apoptosis rate of all groups of cells was analyzed by flow cytometry. ANOVA was used to compare the groups. *p<0.05 vs si-NC group. #p<0.05 vs si-SIRT3 group.
Effects of SIRT3 knockdown on relevant proteins in LoVo/L-OHP cells after DHA treatment
Finally, we used WB to measure the protein expression of the SIRT3/PI3K/AKT signalling pathway proteins, the drug-resistant protein P-gb, and the apoptosis-related proteins cleaved caspase-3, Bax and Bcl-2. As illustrated in Fig. 4, our data demonstrated that transfection with si-SIRT3 resulted in a notable decline in SIRT3 protein expression and increased protein expression of PI3K and AKT (p<0.05).
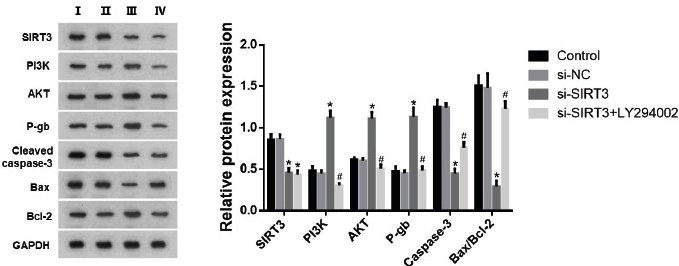
Fig. 4 Effects of SIRT3 knockdown on relevant proteins in LoVo/L-OHP cells after DHA treatment. The protein expression of SIRT3, PI3K, AKT, P-gb, cleaved caspase-3, Bax and Bcl-2 were measured by WB. ANOVA was used to compare the groups. *p<0.05 vs si-NC group. #p<0.05 vs si-SIRT3 group.
On the other hand, treatment with PI3K inhibitor LY294002 remarkably reduced the protein expression levels of PI3K and AKT (p<0.05). Silencing SIRT3 markedly enhanced the expression of P-gb while reducing the protein expression of cleaved caspase-3 and the ratio of Bax/Bcl-2. However, the PI3K inhibitor LY294002 reversed this effect. In the si-SIRT3+LY294002 group, the protein expression of P-gb decreased, while the expression of cleaved caspase-3 and the ratio of Bax/Bcl-2 significantly increased (p<0.05).
DISCUSSION
For researchers, the mechanism of L -OHP resistance in CRC is still elusive. Despite many molecular medicine studies to explain the resistance mechanisms of CRC, it is challenging to distinguish genuinely effective targets for regulating resistance. In addition, most existing multidrug resistance reversal agents have issues such as incomplete resistance reversal, high technical requirements, and significant adverse reactions 20,21. Therefore, finding new sensitizers to combine with L -OHP to treat CRC patients is still necessary. We demonstrated that DHA could inhibit the L -OHP resistance in LoVo human colorectal cancer cells by regulating the SIRT3/PI3K/AKT axis.
Studies conducted in the past have demonstrated that DHA can hinder the growth of cancer cells and trigger apoptosis in pancreatic cancer, ovarian cancer, and colorectal cancer. An added advantage of DHA is its low toxicity towards normal tissue cells 22,23. In addition, DHA also has a killing effect on multidrug-resistant tumor cells 24. In our study, we treated CRC-resistant cells LoVo/ L -OHP with different concentrations of DHA and found that the viability of LoVo/L -OHP cells marked decrease, and the proportion of apoptotic cells increased significantly after DHA treatment. This phenomenon was more pronounced in the group with higher concentrations of DHA, indicating that DHA can inhibit the resistance of LoVo/L -OHP to L -OHP. Similar results have been obtained in other cancers. In non-small-cell lung cancer (NSCLC), DHA can eliminate radiation resistance of NSCLC cell lines and xenograft models by inhibiting the TGF-β, PI3K/Akt, and STAT3 signalling pathways, and enhance the anti-tumor effect of radiotherapy 25. In breast cancer, DHA regulates the STAT3/ DDA1 axis to inhibit the proliferation of breast cancer tumor cells 26. In addition, Yao et al. confirmed that DHA can effectively sensitize CRC cells to 5-fluorouracil (5-FU) by mediating ROS-induced apoptosis 27. Wang et al. also showed that DHA inhibits the characteristics of cancer stem-like cells (CSLCs) in CRC through the AKT/mTOR signalling pathway 28. These results suggested that DHA can be a sensitizing cancer treatment agent.
The protein and mRNA levels of SIRT3 were enhanced in LoVo/L -OHP cells after treatment with DHA, indicating that SIRT3 may be involved in the sensitization of LoVo/ L -OHP by DHA. Cao et al. found that activation of SIRT3 inhibits cisplatin resistance in lung cancer cells and reduces the proliferation and invasion of lung cancer cells 29. Another research showed that the activity of SIRT3 in colon cancer cells can be enhanced by nitric oxide synthase (NOS), which helps to promote cell apoptosis 30. To study the mechanism of action, we silenced SIRT3 and found that the viability of LoVo/L -OHP cells improved, and the proportion of apoptotic cells and apoptotic proteins cleaved caspase-3 and the ratio of bax/bcl-2 decreased significantly, indicating that the knockdown of SIRT3 affected the resistance of LoVo/L -OHP cells to L -OHP after DHA treatment. Furthermore, we confirmed that silencing SIRT3 enhanced the expression of PI3K and AKT in LoVo/L -OHP cells. The aberrant activation of the PI3K/AKT pathway acts against chemotherapy-induced apoptosis by increasing the expression of anti-apoptotic genes (bcl-2 and XIAP) and reducing the expression of pro-apoptotic genes (bax) 31. In our study, we inhibited the PI3K/AKT axis with LY294002 and found that the viability of LoVo/L -OHP cells decreased while apoptosis increased. This also reversed the effect of knocking down SIRT3 on the viability and apoptosis of LoVo/L -OHP cells. Zhang et al.32 found that inhibiting the PI3K/AKT signalling pathway in CRC cells can inhibit cell proliferation and activate apoptosis, thereby reversing oxaliplatin resistance, consistent with our research results.
Limitation
Our research also has some limitations. Whether other genes regulate SIRT3 is not clear. We also did not perform in vivo experiments to confirm the apoptosis results. All these need further studies to illustrate.
Our study found that DHA could inhibit the L -OHP resistance in LoVo human colorectal cancer cells by regulating the SIRT3/ PI3K/AKT axis. The results might provide a new integrated treatment approach for CRC treatment in clinical practice.