INTRODUCTİON
Isoniazid and rifampicin are anti-mycobacterial drugs that are part of the World Health Organisation’s recommended combination treatment regimen for tuberculosis1. Treatment for tuberculosis consists of a quartet of anti-mycobacterial medications, including isoniazid, rifampicin, ethambutol, and pyrazinamide. Furthermore, the isoniazid and rifampicin combination (IRC) includes the supportive phase of tuberculosis treatment 1,2. Although effective in treating tuberculosis, these drugs may cause serious side effects. During treatment, there may be toxic effects on the dermatology, gastrointestinal system, hypersensitivity, neurology, hematology, and renal system 3. Antituberculosis drugs can cause liver toxicity, which can result in the discontinuation of the drug as well as morbidity and mortality 4. A primary antituberculosis drug, isoniazid, has been reported to cause liver injury through oxidative stress 5. The role of lipid peroxidation (LPO) in the pathogenesis of isoniazid-associated oxidative liver injury has been documented 6. Severe oxidative liver damage is believed to be initiated by reactive oxygen species (ROS) 7. According to the literature, isoniazid has hepatotoxic effects due to the formation of the acetylhydrazine metabolite due to its acetylation 1. Although rifampicin alone has a low hepatotoxicity potential 6, it exhibits additive and synergistic hepatotoxicity when used with isoniazid in treating tuberculosis 4. This has been attributed to the stimulation of hydrolases by rifampicin and the formation of hepatotoxic reactive metabolites from isoniazid 8.
The protective effect of thiamine pyrophosphate (TPP), thiamine’s active metabolite, was evaluated in IRC-induced hepatotoxicity in rats 9. Evidence in the literature shows that TPP protects various organs from oxidative and proinflammatory cytokine damage 10-12. Cinnamon, which we also investigate in our study against the possible toxicity of IRC on the liver, is the bark of some Cinnamomum (Lauraceae) species 13. Cinnamon has been shown to have anti-inflammatory, antioxidant, and many other beneficial biological properties in the literature 14. It has been reported that cinnamon protects the heart, liver, kidney, blood, brain, and spleen from the toxicity of chemicals through its antioxidant, radical-scavenging, and LPO-suppressing properties15. The anti-inflammatory effect of cinnamon extract (CE) has been associated with inhibition of proinflammatory cytokines 16. This information suggests that TPP and CE may be helpful in treating IRC-induced liver injury. This study was designed to investigate the protective effects of TPP and CE against IRC-induced liver injury in rats.
MATERİALS AND METHODS
Animals
Twenty-four male albino Wistar rats (282-294 g, 5-6 months old) purchased from the Experimental Animal Research and Application Centre of Erzincan Binali Yildirim University were used in the study. The rats were housed in an environment with appropriate temperature (22±2°C), humidity (50-60%) and 12-h light-dark cycle and fed ad libitum. Experimental procedures were carried out after the approval of the local Animal Experiments Ethics Committee of Erzincan Binali Yıldırım University (Date: 31.08.2023, Decision number: 29).
Chemical substances
Isoniazid and Rifampicin were procured from Koçak Farma Ilaç ve Kimya Sanayi (Istanbul, Turkey), Thiamine pyrophosphate was procured from BioPharma (Moscow, Russia), Cinnamon extract was procured from Solgar (Leonia, USA), and thiopental sodium used for the experiment was procured from IE Ulagay (Istanbul, Turkey).
Experimental groups
The rats (n=6/each group) were randomly divided into healthy control (HG), isoniazid + rifampicin (IRG), TPP + isoniazid + rifampicin (TIRG), and CE + isoniazid + rifampicin (CIRG) groups.
Experimental process
In the experiment, TPP (25 mg/kg) was administered intraperitoneally to the TIRG. CE (100 mg/kg) was administered orally by gavage to the CIRG. In both the HG and IRG, distilled water was administered the same way. To the IRG, TIRG, and CIRG, isoniazid (50 mg/kg) and rifampicin (50 mg/ kg) were given orally one hour after the TPP, CE, and distilled water were given. The indicated treatment protocol was administered once daily for seven days. At the end of the seventh day, blood samples were collected from the tail vein of the rats for alanine aminotransferase (ALT) and aspartate aminotransferase (AST) analyses. The rats were then euthanized with 50 mg/kg thiopental sodium intraperitoneally, and liver tissues were collected. Malondialdehyde (MDA), total glutathione (tGSH), superoxide dismutase (SOD), catalase (CAT), nuclear factor kappa B (NF-κB), tumor necrosis factor-alpha (TNF-α), interleukin 1 beta (IL -1β), and interleukin 6 (IL -6) were analyzed in the liver tissues. Liver tissues were also examined histopathologically.
Biochemical analyses Preparation of the samples
The tissues were cleaned of blood using physiological saline and pulverized by adding liquid nitrogen. Powdered tissues were dissolved in 50 mM phosphate buffer (pH=7.4). For MDA, tGSH, SOD, CAT, NF-κB, TNF-α, IL -1β, and IL -6 analyses, the supernatants obtained after centrifugation were used.
Tissue MDA, tGSH, SOD, and CAT determination
MDA and tGSH levels and SOD activities in tissues were determined by measuring each with Enzyme-Linked ImmunoSorbent Assay (ELISA) kit (product nos. 10009055, 703002, and 706002, respectively, Cayman Chemical Company) according to the instructions. The CAT analysis was conducted according to the method recommended by Goth 17.
Tissue NF-κB, TNF-α, IL-1β, and IL-6 determination
TNF-α, IL -1β, and IL -6 levels were determined with ELISA kits purchased from East-biopharm Co Ltd (China), and NF-κB levels were measured with commercial ELISA kits purchased from SunRed Biological Technology Co. Ltd (China). The analyses were performed according to the kit instructions.
Determination of ALT and AST in serum. Tubes without anticoagulants were used for blood samples, and serum was used for analyses. After centrifugation, the clear filtrate was separated and stored at -80°C. ALT and AST activities were determined spectrophotometrically using a Cobas 8000 autoanalyzer (Roche Diagnostics GmBH, Germany) with kits (Roche Diagnostics).
Histopathological analysis
Tissue samples were fixed in formalin (10%). Subsequently, tissue samples were washed. Tissues were embedded in paraffin after ethanol (70-100%) and xylol procedures. Sections (4-5 µ) were prepared and stained with hematoxylin-eosin (H&E). Sections were photographed and analyzed using a light microscope (Olympus Inc., Tokyo, Japan) and the DP2-SAL firmware program. Histopathological changes in liver tissue were defined as hepatocyte degeneration, Kupffer cell activation, capillary congestion, and the presence of polymorphonuclear cell (PMNL) infiltration. Each section was graded 0-3 for each criterion (0, absent; 1, mild; 2, moderate; 3, severe). The evaluation was performed by a pathologist who was unaware of the study groups’ assignments.
Statistical analyses
The statistical procedures were conducted using “SPSS for Windows, 22.0” statistical software. It was determined that the numerical data were normally distributed by the Shapiro-Wilk test; therefore, one-way ANOVA was used for the analysis. According to the results of Levene’s test, Tukey HSD or Games Howell tests were used as post hoc tests for intergroup comparisons. The biochemical data were presented as mean ± standard deviation (X±SD). The Kruskal-Wallis test was preferred for analyzing semiquantitative histopathologic grading data, and then the post-hoc Dunn’s test was employed for the analysis. Statistical results were presented as median (quartile 1-quartile 3). The significance level was set at p<0.05.
RESULTS
Analysis of MDA, tGSH, SOD and CAT levels in liver tissue
It can be seen from Fig. 1A that MDA levels in liver tissue increased in the IRG group (5.48±0.20) compared to healthy rats (2.39±0.18) (p<0.001). The increase in MDA was observed to be suppressed in the TIRG (2.67±0.24) and CIRG (4.06±0.15) (p<0.001). Compared with CE, TPP suppressed the increase in MDA more effectively (p<0.001). The MDA levels in TIRG and HG were not significantly different (p=0.100).
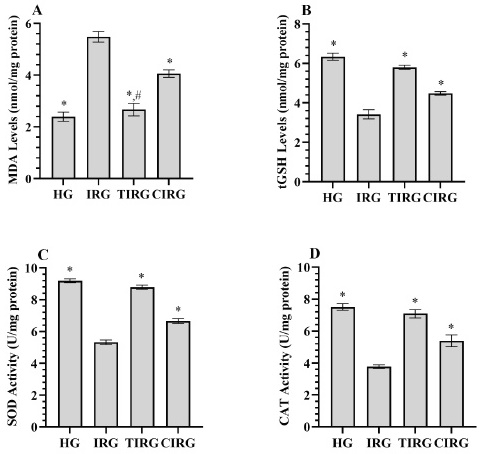
Fig. 1(A-D) Results of analyses of MDA (A), tGSH (B), SOD (C) and CAT (D) data measured from liver tissues. *; p<0.001 vs IRG, # p>0.05 vs HG. MDA: malondialdehyde, tGSH: total glutathione, SOD: superoxide dismutase, CAT: catalase, HG: healthy group, IRG: isoniazid+rifampicin applied group, TIRG: thiamine pyrophosphate+izoniazid+rifampisin applied group, CIRG: Cinnamon extract+izoniazid+rifampisin applied group.
In IRG, a decrease in tGSH levels and SOD and CAT activities (3.42±0.24, 5.33±0.15, 3.78±0.12, respectively) were detected with the increase in MDA compared to HG (6.34±0.18, 9.19±0.12, 7.51±0.21, respectively) (p<0.001). TPP (5.80±0.10, 8.76±0.13, 7.09±0.26, respectively) and CE (4.48±0.09, 6.66±0.16, 5.39±0.37, respectively) inhibited the decrease in tGSH levels and SOD and CAT activities (p<0.001). TPP treatment prevented the decrease in antioxidants more effectively than CE (p<0.001) (Fig. 1B-D).
Analysis of NF-κB, TNF-α, IL-1β and IL-6 levels in liver tissue
As presented in Fig. 2A-D, the levels of NF-κB, TNF-α, IL -1β, and IL -6 in the liver tissues of rats in the IRG group (4.86±0.09, 4.68±0.09, 6.29±0.15, 5.70±0.12, respectively) were higher than those in the HG group (2.38±0.11, 2.13±0.07, 3.37±0.19, 2.49±0.24, respectively) (p<0.001). Compared to the IRG group, both TPP (2.57±0.12, 2.37±0.11, 3.68±0.26, 2.77±0.11, respectively) and CE (3.25±0.15, 3.31±0.14, 5.00±0.48, 4.02±0.22, respectively) inhibited these increases in NF-κB, TNF-α, IL -1β and IL -6 (p<0.05). However, this inhibition was more significant in the TPP than in the CE group (p<0.05). There were similarities between TIRG and HG in terms of NF-κB (p=0.315), IL -1β (p=0.157) and IL -6 (p=0.064) levels.
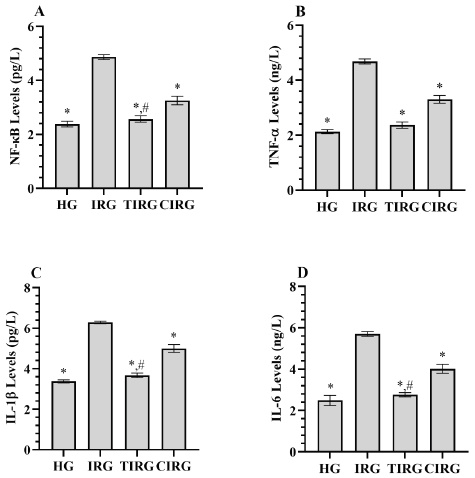
Fig. 2 (A-D) Results of analyses of NF-κB (A), IL -1β (B), TNF-α (C), and IL -6 (D) data measured from liver tissues. *; p<0.05 vs IRG, #, p>0.05 vs HG. NF-κB: nuclear factor kappa-B, IL -1β: interleukin 1-beta, TNF-α: tumor necrosis factor-alpha, IL -6: interleukin-6, HG: healthy group, IRG: isoniazid+rifampicin group, TIRG: thiamine pyrophosphate+izoniazid+rifampisin group, CIRG: Cinnamon extract+izoniazid+rifampisin group.
Analysis of ALT and AST activities in serum samples
As presented in Fig. 3A-B, ALT and AST activities measured from the serum of rats in the IRG (86.67±4.55, 220.00±7.72, respectively) were found to be increased according to HG (29.17±4.02, 39.17±4.92, respectively) (p<0.001). The increase in ALT and AST activities in the IRG appeared to be suppressed in TIRG (32.83±3.55, 45.83±4.62, respectively) and CIRG (56.50±2.43, 92.50±4.51, respectively) (p<0.001). TPP inhibited this increase in ALT and AST activities more effectively than CE (p<0.001). Serum ALT (p=0.165) and AST (p=0.200) activities of TIRG and HG were similar.
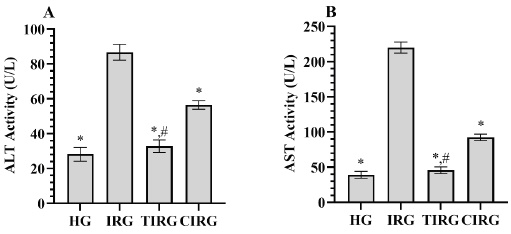
Fig. 3 (A-B) Results of analyses of ALT (A) and AST (B) data measured from serum. *; p<0.001 vs IRG, #, p>0.05 vs HG. ALT: alanine aminotransferase, AST: aspartate aminotransferase HG: healthy group, IRG: isoniazid+rifampicin group, TIRG: thiamine pyrophosphate+izoniazid+rifampisin group, CIRG: cinnamon extract+izoniazid+rifampisin group.
Histopathologic findings
There were normal central arteries in the liver tissue sections, radially arranged hepatic cords in the lobules, and normal tissue structure in the HG group, as presented in Fig. 4A and Table 1.
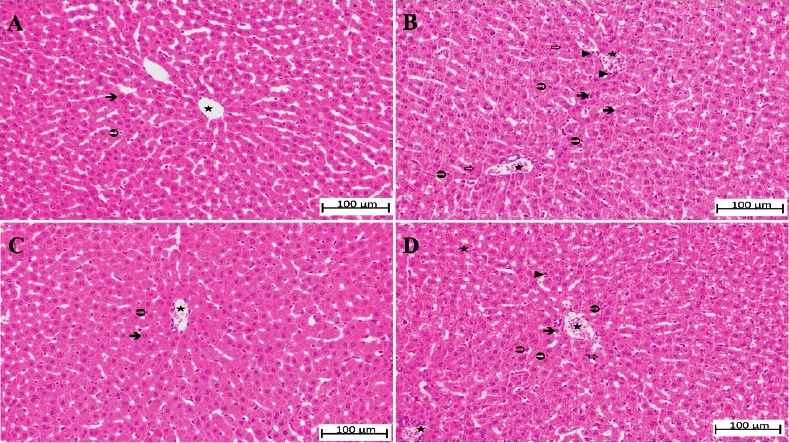
Fig. 4 (A-D) A. Liver tissue belonging to the HG. ; hepatocyte,
; Kupffer cell,
; normal appearance of blood vessels (H&E x200). B. Liver tissue belonging to the IRG.
; degenerated hepatocyte,
; irregularity in hepatic cords,
; increased Kupffer cells, ►; polymorphonuclear cell infiltration,
; moderately congested blood vessel (H&E x 200). C. Liver tissue belonging to the TIRG.
; hepatocyte with normal morphology,
: Kupffer cell,
; slightly congested blood vessel (H&E x 200). D. Liver tissue belonging to the CIRG.
; moderately degenerated hepatocytes,
; increased Kupffer cells,
; irregularity in the hepatic cords, ►; weakly polymorphonuclear cell infiltration,
; moderately congested blood vessels (H&E x200). HG: healthy group, IRG: isoniazid+rifampicin group, TIRG: thiamine pyrophosphate+izoniazid+rifampisin group, CIRG: Cinnamon extract+izoniazid+rifampisin group.
Table 1 Analysis results of histopathological grading data of liver tissues from rats.
Parameters | Groups (n=6/each groups) | H / p values | |||
---|---|---|---|---|---|
HG (n=6) | IRG (n=6) | TIRG (n=6) | CIRG (n=6) | ||
Median (quartile 1-quartile 3) | |||||
Degeneration | 0(0-0)* | 3(2-3) | 0(0-0)*,# | 2(2-2)£ | 129.013<0.001 |
Kupffer cell activation | 0(0-0)* | 3(2-3) | 0(0-0)*,# | 2(2-2)£ | 129.151/<0.001 |
Congestion | 0(0-0)* | 2(2-3) | 0.5(0-1)*,# | 2(2-2)£ | 122.132/<0.001 |
PMNL infiltration | 0(0-0)* | 3(3-3) | 1(1-1)* | 1(0.25-2)* | 114.468/<0.001 |
Histopathological grading (0-3): 0: absent, 1: mild damage, 2: moderate damage, 3: severe damage. * p<0.001 vs. IRG, #; p>0.05 vs. HG, £; p>0.05 vs. IRG, HG: healthy group, IRG: isoniazid+rifampicin group, TIRG: thiamine pyrophosphate + izoniazid + rifampisin group, CIRG: Cinnamon extract + izoniazid + rifampisin group. Statistical analysis was done using the Kruskal-Wallis test. Then, Dunn’s test was applied. Data are presented as median (quartile 1-quartile 3). p<0.05 was considered significant.
Upon examination of the liver tissue of the IRG group, it was observed that the hepatocytes around the central artery had lost their typical morphology, were degenerating, their nuclei bulged and appeared pyknotic on occasion. A noticeable phenomenon was the loss of radial arrangement of hepatic cords, which resulted in irregular and spiralized cords. There was a marked and moderate degree of congestion in both the central artery and surrounding blood vessels. A significant increase was observed in the number of Kupffer cells. Many PMNLs were detected infiltrating the pericapillary area (Fig. 4B and Table 1).
Notably, in the TIRG group treated with TPP, the arrangement of hepatic cords and hepatocytes was generally normal, and the Kupffer cell population was relatively similar to the control group. PMNL infiltration was rare throughout the tissue (Fig. 4C and Table 1).
In the CE-treated CIRG group, it was remarkable that hepatic cords were irregular, and hepatocyte degeneration was at a moderate level. In general, it was observed that the congestion in the blood vessels was at a moderate level, and the Kupffer cell population was increased. Mild PMNL infiltration was also determined in the pericapillary area (Fig. 4D and Table 1).
DİSCUSSİON
The primary treatment protocol for tuberculosis consists of isoniazid, rifampicin, pyrazinamide, and ethambutol for six months 18. Increased dosages of these drugs have been tried to overcome resistance, which has increased the incidence of side effects 19. Drug-related side effects often result in a change in treatment, which can adversely affect the treatment’s effectiveness. One of the most common side effects of anti-tuberculosis treatment is hepatotoxicity 18. In the literature, mitochondrial dysfunction and oxidative stress have been reported to be involved in the mechanism of IRC-induced hepatotoxicity 19. In this study, TPP 10 and CE 14, both with antioxidative activity, were examined biochemically and histopathologically for their protective effects on IRC-induced hepatotoxicity. Our biochemical analysis revealed that IRC use caused an increase in oxidant (MDA) and inflammatory markers (NF-κB, TNF-α, IL -1β, and IL -6), a decrease in antioxidants (tGSH, SOD, and CAT), and an increase in ALT and AST activities.
As mentioned above, the role of LPO in the pathogenesis of isoniazid-induced oxidative liver injury was demonstrated 6. Rifampicin, on the other hand, was reported to exhibit additive and even synergistic hepatotoxicity when used in combination with isoniazid 4. The cause of this condition has been attributed to rifampicin stimulating hydrolases and the formation of hepatotoxic reactive metabolites from isoniazid 8. As is well known, MDA is an LPO product used as a biomarker of oxidative stress 20. MDA exhibits a toxic effect; additionally, converting ROS into active substances causes cell membrane damage, leading to apoptosis and liver necrosis 21. An experimental study has found that four weeks of IRC treatment has increased MDA in rat livers 22. By suppressing the increase in MDA in the group administered TPP in conjunction with IRC, it is evident that TPP displays antioxidant properties. It was suggested in the literature that thiamine interacted with free radicals and hydroperoxides and inhibited LPO 23. Previously, TPP was also tested in oxidative liver injury induced by propofol 11 and metazimol 24 and was found to inhibit the increase in MDA levels in rat livers, similar to our study. In our study, CE suppressed the increase in MDA levels. However, CE suppressed the increase in MDA levels less than TPP. Moselhy et al. reported that aqueous and ethanol extracts of cinnamon could prevent the increase in MDA levels by reducing LPO in carbon tetrachloride-induced oxidative liver injury in rats 25.
The liver tissues of the animals were also analyzed in terms of antioxidants. For this purpose, tissue tGSH levels and SOD and CAT activities were determined. GSH represented the most important endogenous non-enzymatic antioxidant. GSH plays a role in the protection of cells from ROS.10. The literature reported that hydrazine reacted with the sulfhydryl group of GSH, depleting its levels in hepatocytes and causing cell death 26. In our study, tGSH levels were found to be decreased in the IRC group. Similarly, Zhang et al. found that IRC decreased GSH levels in liver tissue and serum samples 27. During the metabolism of antituberculosis drugs, toxic metabolites and free radicals deplete endogenous antioxidants, making the liver susceptible to further damage 28. According to the literature, external administration of agents with antioxidant activity could contribute to tissue defense in such cases 29. Our study determined that TPP administered with IRC prevented the decrease in tGSH levels and even maintained them at healthy levels for the healthy group. Delen et al. similarly concluded that TPP inhibited the depletion of tGSH in the liver caused by propofol use 11. The decrease in tGSH levels in CE-treated animals was suppressed, although not as much as in TPP-treated animals. In a previous study, CE was found to possess antioxidant properties and to suppress GSH depletion in liver tissue 29.
Results of our analysis indicated that SOD and CAT activities were also decreased in the IRC group in which tGSH levels were low. By accelerating the conversion of oxygen to hydrogen peroxide (H2O2), SOD was the first antioxidant enzyme to fight ROS 27. CAT, on the other hand, converted the toxic H2O2 into H2O and oxygen 30. As a result, SOD and CAT acted as mutually supportive antioxidant enzymes that protected ROS 27. It was demonstrated in previous preclinical studies that IRC decreased the activity of SOD and CAT in the liver 31,32. The results of this study revealed that TPP treatment was able to prevent the decline in SOD and CAT activities. Throughout the body, TPP catalyzes various chemical reactions. As a cofactor of enzymes involved in maintaining cell redox, it synthesized reduced nicotinamide adenine dinucleotide phosphate and glutathione and increased the synthesis of antioxidants 24. In addition, Bedir et al. found that TPP prevented metamizole-induced decreases in the activities of SOD and CAT in the liver tissues of rats 24. El-Kholy et al. used CE, another therapeutic drug, to treat oxidative liver damage induced by amoxicillin/clavulanate and found that it inhibited the decrease in SOD and CAT activity, similar to our results 29.
Previously, it was reported that IRC treatment caused inflammation. This leads to activation of macrophages and infiltration of circulating immune cells into the tissue 28. In contrast, sterile inflammation also contributed to hepatotoxicity by increasing oxidative stress. NF-κB was a transcription factor that modulated inflammation 28,33. According to our biochemical results and previous studies, increased NF-κB levels with IRC treatment confirmed inflammatory activity 28,31. In the TPP-treated group, the increase in NF-κB levels seemed to be prevented. Similarly, Ozer et al. found that TPP suppressed the increase of NF-κB in damaged ovarian tissue 34. In our CE-treated group, NF-κB levels were higher than the TPP group and lower than the IRC group. A recent study found that this increase was suppressed in obese rats treated with Cinnamon powder compared to rats with increased hepatic NF-κB expression with obesity 35. Our study also investigated liver tissues for the levels of proinflammatory cytokines such as TNF-α, IL -1β, and IL -6.
Increased ROS released inactive NF-κB through a series of reactions. Activated NF-κB induced gene transcription of TNF-α and IL -1β 21. On the other hand, TNF-α, which is considered the primary mediator inducing the inflammatory response, has been reported to activate NF-κB by binding to the TNF receptor 31,36. In addition, it was pointed out that TNF-α increased ROS production that triggered liver injury through various pathways 31. IL -1β had intense proinflammatory activity like TNF-α 37. Although IL -6 also prevented acute liver inflammation, its prolonged stimulation resulted in tissue damage to the liver 38. Our study discovered that TNF-α, IL -1β, and IL -6 levels were similarly increased in the IRC group in which NF-κB levels were increased. Patel et al. also found an increase in TNF-α, IL -1β, and IL -6 levels in their study on the effects of IRC on the liver 38. Cytokine levels in rats administered with TPP and CE were lower than those of the IRC group. No studies have been conducted in the literature on the effect of TPP on tissue cytokine levels in oxidative liver injury. However, it was reported that TPP prevented the increase in TNF-α and IL -1β levels due to ethanol toxicity in optic nerve tissue 39. In the literature, studies also reported that CE, another therapeutic agent, exhibited anti-inflammatory activity by suppressing the increase in TNF-α, IL -1β, and IL -6 levels induced by various causes in the liver 40,41.
In this study, the ALT and AST activities of blood samples obtained from animals were measured. These transaminases were sensitive markers of liver cell damage, and these biomarkers have been widely used in the past and present to assess liver function 42. In both clinical and experimental studies, it has been demonstrated that IRC treatment increases the levels of ALT and AST 18,26,42. Our study also demonstrated increased ALT and AST activity after IRC treatment. A lower enzyme activity was observed in both TPP and CE-treated groups, but a lower activity was observed in the TPP-treated group. As previously reported, TPP inhibited the increase in ALT, AST, and lactate dehydrogenase activities following acetaminophen 43 and cisplatin-induced liver injury 12. Mosely et al. also demonstrated that CE inhibited the increase in ALT and AST activities 25.
A histologic analysis of liver tissues confirmed our biochemical findings. Degeneration of hepatocytes and marked congestion of blood vessels were observed in the IRC group. In addition, an increase in the number of Kupffer cells and PMNL was observed. According to previous studies, IRC induced changes in the liver, such as sinusoidal dilatation, infiltration of immune cells, inflammation in the portal system, and necrosis of the liver 27,28. Our histopathological analysis showed that the hepatocytes and Kupffer cell populations of the TPP-treated group were similar to those of the control group, and rare evidence of PMNL infiltration was observed. Several studies demonstrated that TPP protected liver tissue from oxidative damage and prevented structural changes such as cellular degeneration, necrosis, and infiltration of inflammatory cells 11,43. In the CE group, there was moderate hepatocyte degeneration and vascular congestion. In addition, there was a moderate increase in the Kupffer cell population and mild PMNL infiltration. However, some previous studies reported that CE treatment altogether 44 or almost entirely 25 prevented tissue damage in oxidative liver injury. However, our histopathologic evaluation indicated that TPP protected the histologic structure of the liver better than CE, similar to our biochemical results.
Limitations
In the future, total oxidant levels and antioxidant levels should be measured to investigate the mechanism of hepatoprotective effect of TPP and CE in greater detail. Additionally, we believe that anti-inflammatory cytokine levels should be evaluated.
Our results revealed that IRC induced an increase in oxidants and proinflammatory cytokines and a decrease in antioxidants in the liver tissues of the animals. Moreover, IRC treatment caused an increase in liver function parameters and damage to the histological structure of the liver. TPP and CE inhibited both biochemical and histopathologic changes. However, TPP protected liver tissue from IRC-induced damage better than CE. The results of our study indicated that adding TPP to the treatment might be an effective therapeutic strategy for preventing IRC-induced hepatotoxicity. Moreover, based on the results of our literature review, our study was the first to compare the antioxidant and anti-inflammatory properties of TPP and CE. As a result of our current study, we are likely to contribute to future experimental and clinical studies on diseases where oxidative stress and inflammation are involved in the pathogenesis.